20 July 2020
PDF version [539KB]
Professor
Andrew Blakers and Dr Matthew Stocks
Australian National University
Executive
summary
- Solar energy supply is dilute, but vast, widespread and
indefinitely sustainable. Its utilisation for electricity generation typically has
minimal environmental, social and security impacts over unlimited time scales.
Photovoltaics (PV) are currently the leading energy generation technology in
terms of annual net global deployment rates. Intermittency of supply can be
offset by energy storage systems such as Pumped Hydro Energy Storage systems
and strengthening the connectivity of the grid.
Australian electricity options are short briefings on the principal energy sources
and storage options being debated in Australia, including: coal, natural gas,
wind, nuclear, photovoltaics (PV) and pumped hydro energy storage (PHES).
The global COVID-19 pandemic and its economic
consequences mean that statements and projections about future demand and
pricing of energy options may no longer be reliable. Readers should note that
some figures quoted in these briefings may pre-date the pandemic. |
Photovoltaic technology
PV is an elegant technology for the direct production of
electricity from sunlight without moving parts. Sunlight is absorbed by the
solar cell and the solar power is converted to electrical power with a typical
conversion efficiency of around 20% (the remaining solar power (80%) is either
reflected or becomes heat and is discarded). This process of conversion is
called photovoltaics (photo=light, voltaics=voltage).
A solar cell is typically made from a square wafer of
silicon (156 millimetres square and about one-sixth of a millimetre thick).
Sunshine absorbed by the silicon detaches electrons from their host silicon
atoms. Near the sunward surface is a ‘one-way membrane’ called a pn-junction.
The pn-junction is formed by diffusing tiny quantities of phosphorus into the
wafer to a depth of about one micrometre. When a free electron crosses this
junction it cannot easily return, causing a negative voltage to appear on the
sunward surface (and a positive voltage on the rear surface). Metal electrodes
are printed on both surfaces of the solar cell. The sunward and rear electrodes
are connected via an external circuit to extract current, voltage and power
from the solar cell.
Solar cells are packaged into a solar module, also known as
a PV panel, to protect them from the environment. Modules typically comprise
60-72 silicon solar cells contained within transparent plastic behind thick
glass to form a durable PV module. The package usually includes aluminium
edge-frames to help with module mounting in the field or on a rooftop. A
junction box is added to the rear to house the electrical terminals.
This package is extremely reliable with PV modules now
warranted for up to 30 years. PV modules have high reliability and low
maintenance cost due to the lack of moving parts. Deterioration of PV modules
can be caused by physical destruction caused by human action or violent
hailstorms, slow chemical changes leading to yellowing of transparent
encapsulation materials and ingress of moisture causing corrosion of metallic
components.
A 20% efficient PV module will yield 200 watts of power per
square metre of module at noon on a sunny day. On a sunny day where the amount
of solar energy received is equivalent to 5 hours of noon-equivalent sunshine,
the module would produce 1000 watt-hours of energy per square metre, which is
one kilowatt-hour (kWh) per square metre.
Electricity produced in the PV module is conducted to a
power conditioning unit that optimises voltages, converts the direct current
produced by the solar cells to the alternating current used in electrical
grids, transforms the voltage to match that of the local grid, and manages
interfacing with the local grid.
Most (95%) of the world’s PV market is serviced by
crystalline silicon solar cells. This is because silicon has important
advantages over other potential PV materials, including abundance (silicon is
the second most abundant element in the Earth’s crust), low cost, non-toxicity,
high efficiency, device performance stability, the highly advanced state of
knowledge of silicon material and technology, and the advantages of incumbency.
The latter comprises extensive and sophisticated supply chains, large-scale
investment in mass production facilities, deep understanding of silicon PV
technology and markets, and the presence of thousands of highly trained silicon
specialists—scientists, engineers and technicians.
Despite producing zero-emission electricity, photovoltaics
can create some
relatively small environmental impacts in their broader lifecycle,
including during the extraction of some mineral inputs, their manufacture, and
at end-of-life. The International
Renewable Energy Agency has projected that PV panels could account for 78
million tonnes of annual waste by 2050 and has looked at opportunities for
recycling or repurposing these products. This is far less than the waste
associated with fossil and nuclear energy.
Figure 1: Royalla 20 MW solar farm near Canberra showing
thousands of PV modules mounted on support frames. The aluminium frame
surrounding each module can also be seen, as well as the 72 cells packed into
each module.
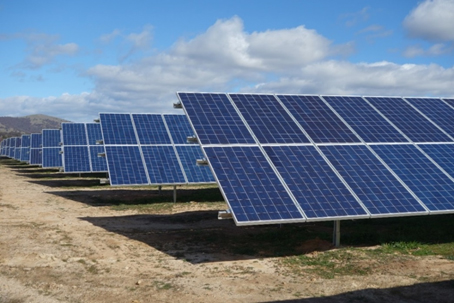
Source: A. Blakers
PV systems
Large numbers of PV modules can be used to create PV
systems. Some PV systems are mounted on fixed support structures that are
tilted up to face the equator, with a tilt equal to the angle of latitude.
Increasingly, large scale PV systems use sun-trackers to maximise annual
output.
Alternatively, PV systems can be mounted on the roofs of
houses and commercial buildings. A typical ground-mounted PV system has a peak
power output of 100–1,000 Megawatts (MW). A typical new domestic PV system has
a power capacity of 5–10 kilowatts (kW), while a commercial building might host
a multi-Megawatt system.
Australia has the highest uptake of rooftop solar in the
world. By mid-2019, there were more
than two million PV systems registered with the Clean Energy Regulator in
Australia with a combined capacity of more than 9,000 MW.
PV is unusual in that the unit cost of energy is similar for
large (MW) and small (kW) systems—large systems have lower capital costs but
higher financing costs and vice versa. Virtually all other energy sources have
strong diseconomies of scale with small size. This confers a major advantage on
PV, since it has markets at every scale from watts to gigawatts for the same
basic product—the silicon solar cell.
The versatility of PV has contributed to its history of sustained
growth. The average annual growth rate over the past 25 years has been 37%
per year. PV has now emerged as the world’s fastest growing generation
technology in terms of annual net new generation capacity, as shown in Figure
2. PV is approaching half of net new generation capacity installed each year,
with coal, oil, gas, nuclear, wind, hydro and other renewables providing the
balance. The fundamental reason is low and falling PV prices.
Figure 2: New global generation capacity added in 2015-2019
by technology type. PV is growing rapidly whilst the other generation
technologies have negligible growth in annual net new deployment.
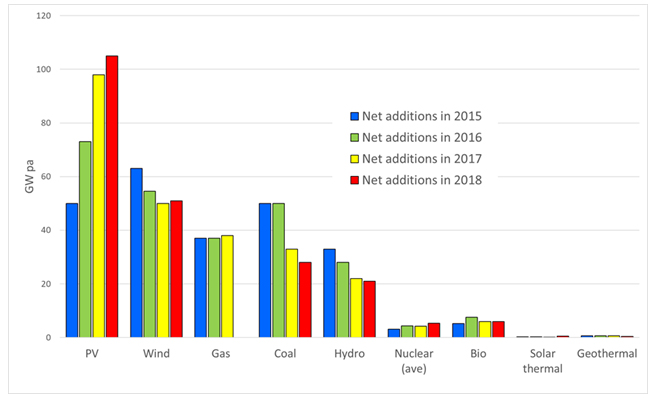
Source: REN21 2015, UNEP 2014, IRENA 2016
In earlier decades, PV found widespread use in niche markets
such as consumer electronics, remote area power supplies and satellites.
Throughout the world, remote area energy solutions are based upon various
combinations of PV, wind, diesel and batteries. In recent decades the industry
has expanded and costs have declined very rapidly.
PV module prices have been falling rapidly for decades. This
is caused by steadily improving technology and the benefits of mass production.
The PV
learning curve illustrates the module price reduction achieved for each
doubling of global cumulative production. All learning curves eventually
flatten out as the technology matures and reaches the bottom of its cost curve.
However, it is clear that PV is far from reaching the bottom of its price
curve. The cost of silicon PV modules is likely to continue to decline for many
more years.
The US investment bank Lazard has published
a report showing current global PV costs can be as low as US$36/MWh,
depending upon the scale of the installation, solar intensity, financial
parameters and local factors. This is lower than competing new-build fossil,
nuclear and renewable technologies in most parts of the world, and prices
continue to fall.
Australia is experiencing a remarkable renewable energy
transition. Over the three years 2018-20, about 17 Gigawatts (GW) of new wind
and solar PV electricity generation systems are being completed, most of it in
the National Electricity Market (NEM). For perspective, the average and peak
electricity generation in the NEM is about 23 and 35 GW respectively. This 17
GW equates to 200-250 Watts of new renewable energy per person per year
compared with about 50 Watts per person per year for the European Union (EU),
Japan, China and the USA (see Figure 3).
Figure 3: Annual per capita renewables deployment rate
for countries and regions.
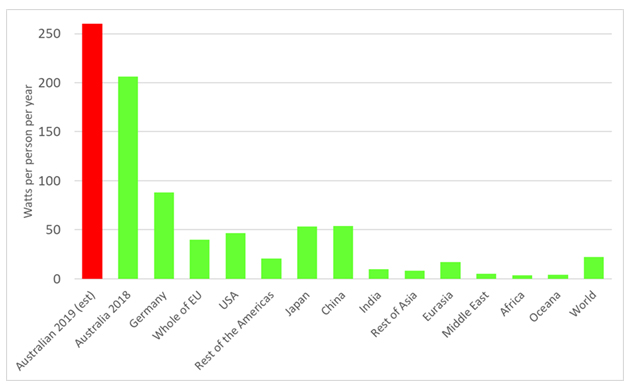
Sources: Data for Australia (2018 and 2019) is from the
Clean Energy Regulator and data for other countries/regions (2018) is from
IRENA.
Curtailment of global greenhouse
gas emissions
Use of coal, oil and gas for
energy generation causes about 80% of greenhouse gas emissions, with
agriculture contributing most of the rest. To curtail global warming, fossil
fuel use needs to be replaced. PV is the leading contender for such replacement
in electricity generation because of the following attributes:
- The solar resource is very large and ubiquitous. Sunlight will be
available for billions of years to come. Most of the world’s population lives
at low latitudes (less than 35°), where sunlight is abundant and varies little
between seasons. A large proportion of PV systems are installed on rooftops and
in arid regions, thus minimising competition with food production and
ecosystems.
- PV has minimal greenhouse gas emissions and other environmental
impacts and does not need water to operate.
- PV utilises abundant raw materials effectively in unlimited
supply—silicon, oxygen, hydrogen, carbon, aluminium, glass, steel and small
amounts of other common materials.
- There are minimal security concerns in respect of warfare,
terrorism and accidents. More widely distributed PV generation across the world
could reduce the risk of wide-scale electricity generation infrastructure disruption
from natural disasters, war and terrorism.
- PV is already low cost and in mass production.
- Wind energy is an important adjunct to PV. However, other clean
energy technologies can realistically play only a minor supporting role. Solar
thermal and nuclear generators are being deployed at far smaller rates than the
fast-growing PV industry (because of higher costs), and extravagant growth
rates would be required to achieve the same new capacity installation rates as
PV. Hydro power, geothermal, wave and tidal energy are only significant
prospects in some regions, and biomass has very low solar conversion efficiency
which means that severe conflict will arise with food production and ecosystems
for land and water if used on a large scale.
Issues with
PV
The first is the intermittency of supply—electricity isn’t
generated when the sun isn’t shining, either at night or in bad weather, with
the associated challenge of matching the generated PV power to meet energy
demand where the two do not coincide. There are three main solutions to the
‘intermittency problem’: rapidly dispatchable power from energy storage systems
such as pumped hydro (see separate article); power from systems that can
rapidly come online such as gas powered turbines, or creating a more
interconnected grid that has the flexibility to balance supply and demand from
a wider area by smoothing out localised weather events. Sufficient storage or
other generation technologies are required to meet electricity demand at night.
Second, there is a need to maintain frequency stability in
the grid in the face of significant changes to production and demand, which is
generally done by the heavy rotating machinery of base-load power stations.
This frequency stabilisation can be performed by other paired technologies that
can respond to fluctuations within seconds, such as large batteries (as the
Tesla battery has done at Hornsdale
in South Australia), by hydroelectric systems or even hydrogen electrolysis
generators.
Emerging technologies
Silicon solar cells are by far the largest generation
technology in terms of annual net global capacity additions. A promising
development in PV is perovskites in solar
cells instead of, or as well as, silicon. Perovskite refers to a class of
lab-grown, crystalline materials that mimic the structure of the naturally
occurring mineral perovskite. They could be relatively cheap, are highly
efficient at converting sunlight to electricity, can be used in a thin-film
format and offer other potential
benefits. However, there are serious concerns about performance stability,
and they are not yet in commercial production.
References
and further reading
A Blakers, B Lu and M Stocks, ’100% renewable electricity
in Australia’, Energy, Volume 133, pp. 471-482, August 2017.
Clean Energy Council, Clean
Energy Australia report 2020.
For copyright reasons some linked items are only available to members of Parliament.
© Commonwealth of Australia

Creative Commons
With the exception of the Commonwealth Coat of Arms, and to the extent that copyright subsists in a third party, this publication, its logo and front page design are licensed under a Creative Commons Attribution-NonCommercial-NoDerivs 3.0 Australia licence.
In essence, you are free to copy and communicate this work in its current form for all non-commercial purposes, as long as you attribute the work to the author and abide by the other licence terms. The work cannot be adapted or modified in any way. Content from this publication should be attributed in the following way: Author(s), Title of publication, Series Name and No, Publisher, Date.
To the extent that copyright subsists in third party quotes it remains with the original owner and permission may be required to reuse the material.
Inquiries regarding the licence and any use of the publication are welcome to webmanager@aph.gov.au.
This work has been prepared to support the work of the Australian Parliament using information available at the time of production. The views expressed do not reflect an official position of the Parliamentary Library, nor do they constitute professional legal opinion.
Any concerns or complaints should be directed to the Parliamentary Librarian. Parliamentary Library staff are available to discuss the contents of publications with Senators and Members and their staff. To access this service, clients may contact the author or the Library‘s Central Entry Point for referral.